A look into how base editors operate and their importance
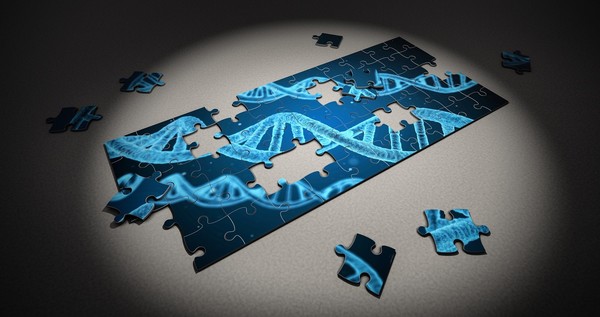
HUMAN GENETIC disorders can be caused by more than 30,000 mutations in the human genome. These particular mutations are caused by point mutations, when a single nitrogenous base is changed, inserted, or deleted in the DNA sequence. Clustered regularly interspaced short palindromic repeats-CRISPR associated protein 9 (CRISPR-Cas9), one of the most predominantly used gene-editing tools, is inefficient when it comes to editing point mutations. Therefore, scientists have turned to base editors as a potential solution; base editors are capable of editing single points of mutation in the DNA more precisely. So far, testing on animal models with genetic disorders similar to those seen in humans has yielded optimistic results that suggest that base editors will be equally as effective in humans. Furthermore, the continued development and modification of these editors have increased their potential to correct nearly all genetic point mutations in humans.
The basics of genetics and mutations
Point mutations in genetic disorders are caused when one of the four chemical bases in DNA is altered, which causes the specific pairing of bases on the gene to change as well. A DNA molecule consists of nucleotides, which are comprised of a phosphate group, a sugar group, and a base. These nucleotides are arranged to form two strands that are twisted around in a structure known as a double helix. The four chemical bases in DNA are adenine (A), guanine (G), cytosine (C), and thymine (T). The bases in the top strand have to correspond to the bases in the bottom strand. In DNA, four pairings of these chemical bases are possible: adenine-thymine (A-T), cytosine-guanine (C-G), thymine-adenine (T-A), and guanine-cytosine (G-C). Mistakes during DNA replication can lead to an insertion or deletion of a base in a given DNA sequence that changes the original pairing; a divergence from a given sequence of pairings can lead to genetic diseases like sickle-cell anemia.
For sickle-cell anemia, a single mutation in a key gene leads to abnormal protein production; in this gene, the DNA sequence in the top strand, C-T-C, is paired with the sequence of G-A-G in the bottom strand. However, imagine if the top strand’s sequence was altered to C-A-C; this is a classic example of a point mutation. Now, the pairing in the bottom strand sequence still has to match the top strand even if it does contain a mutation, and it does so with the sequence of G-T-G. This point mutation affects hemoglobin, a protein that oxygenates the body, and leads to health complications such as strokes, swelling in arms and legs, and lack of blood flow to the brain.
The journey to even thinking about curing a detrimental genetic disorder like sickle-cell anemia starts with, surprisingly, bacteria. The CRISPR-Cas system is a type of immune system naturally found in bacteria and was discovered after scientists observed that bacteria performed self-defense by simply cutting the DNA of the invading virus. CRISPRs are sequences that “remember” the DNA sequence of an invading virus. These CRISPR sequences help identify the same virus if it invades the bacteria again. When it does, the CRISPR sequence “recalls” the DNA of the invader and quickly locates it the second time it invades. The Cas proteins identify and cut the virus, which degrades the whole virus.
Based on this natural system found in bacteria, scientists have developed technology known as the CRISPR-Cas9 system, which uses the same basic concept as a bacteria’s immune system. To edit a gene, a “guide” is engineered to direct the Cas9 protein to the target gene. A normal sequence of the gene is also inserted in order to act as a template to “repair” the mutation in the target sequence. The “guide” finds the target gene and binds to it. Both strands of the DNA are cut by the Cas9 protein. After the Cas9 protein cuts the strands of the target DNA, it is “fixed” at the point where it was cut by using the normal DNA sequence as a template.
Despite what some sensational article titles may imply, base editors are not a new “invention.” In the same vein, the CRISPR-Cas9 system is not old news because these base editors actually use the basic components of CRISPR-Cas9 to operate and improve their precision when editing genetic disorders. Exact precision is needed to correct point mutations, something that CRISPR is unable to provide, so scientists have turned their attention to base editors as a potential solution.
From the lab to trials
David Lui, a professor at Harvard University, made indispensable contributions to the field of gene editing [1]. In 2013, he, along with a few of his colleagues, co-founded Editas Medicine. Three years later, this company was the first biotechnology-based company to go public and was a pioneer in developing gene therapy techniques using CRISPR-Cas technology, which, at the time, was a scientific breakthrough. Perhaps, the only people with more impressive resumes than Lui were his postdoc students, Alexis Komor and Nicole Gaudelli. These two are credited with the “invention” of base editors. On a seemingly ordinary day at the lab, Komor and Gaudelli “invented” base editors— which are capable of precisely editing point mutations.
Just as the CRISPR-Cas system was based on the immune system of bacteria, base editors were built on the backbone of CRISPR. Base editors cut the targeted base of the DNA sequence and replace it with the desired base to fix genetic point mutations. These editors reach the genome through an “empty” virus shell that is injected into the animal or human body. Once a base editor enters the genome, it converts one of the four incorrect bases that causes the genetic disorder into the correct base without breaking the bonded, two strands of the DNA [2]. Unlike CRISPR, base editors do not cut the strands of DNA when they are correcting base-pairing errors, which leads to fewer “off-target” editing. Simply put, CRISPR cuts DNA but cannot control what happens to it afterward. Base editors smoothly swipe out the wrong base for the correct one without any further complications [3].
This breakthrough led Lui to establish Beam Therapeutics, a company with one sole focus: improve gene therapy techniques using base editors [4]. After the genesis of base editors, Komor and Gaudelli turned their focus to developing specific types of base editors: the cytosine-to-thymine base editor (CBE) was Komo’s success story while the adenine-to-guanine base editor (ABE) was Gaudelli’s design after months of trial and error stimulations in the lab [5]. The CBE can convert C-G pairs to T-A ones, and the ABE can convert A-T base pairings to G-C ones. These two types of base editors have the potential to correct almost all genetic mutations in humans.
ABEs have recently been used to cure Hutchinson-Gilford progeria syndrome (HGPS) in a mouse model, a genetic disorder typically seen in children that causes them to age rapidly [6]. Of the mutations in the organs, 20% to 60% of the mutations were altered to the correct base pairing. The ABE had successfully swapped the detrimental A-T pairing for the accurate pairing of C-G. If a genetic disorder in a mouse can be cured by base editors, then the same results have the potential to be replicated in humans.
Even given how precise base editors are when compared to CRISPR, scientists were still not satisfied with the accuracy levels of CBEs. In order to increase their precision, scientists developed APOBEC3G base editors (A3G-BEs), which are 6,000 times more accurate than their predecessors. A3G-BEs, unlike CBEs, reduce the chances of “off-target” editing, meaning they will only edit the target base. This is extremely important because T-to-C mutations account for 38% of known genetic disorders, so preventing the alteration of nearby cytosine bases is extremely essential in curing genetic mutations. This advancement has the potential to precisely correct 540 point mutations in humans.
* * *
Just as improvements to CBEs led to the “invention” of A3G-BEs, base editors can always be modified for more precision. As these base editors have become more precise in their correction of base mutations, their potential for curing genetic disorders has become within reach. While human application and clinical trials of base editors may take years to achieve, given the inherent differences between humans and mice, scientists are confident that clinical trials are next on the agenda given the breakneck speed of base editor development and encouraging experimental results.
[1] Genetic Engineering and Biotechnology News
[2] Nature
[3] Nature Biotechnology
[4] Harvard Gazette
[5] PubMed
[6] Genetic Engineering and Biotechnology News