The role of microscopic robots in advancing medicine
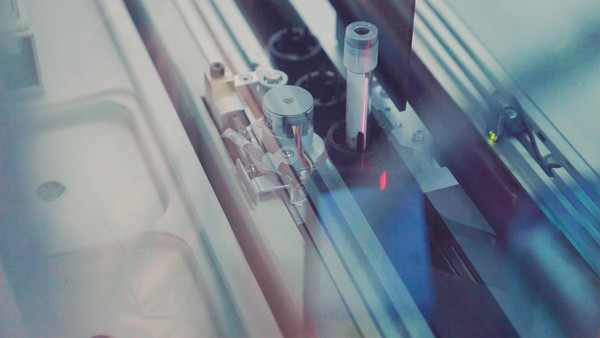
TECHNOLOGY IS becoming increasingly incorporated into biology. After wearable devices such as Virtual Reality (VR) headsets, fitness trackers, and smart glasses, technology and biology are now progressing towards the “embeddable phase,” where digital technology is applied by being implanted into the human body[1]. While embeddable technology like the artificial cardiac pacemaker[2] is already in active use, nanobots are anticipated to further advance the field of medicine. Today, scientists are designing robots with sizes smaller than a human cell at a molecular level for various functions including drug delivery, surgery, medical imaging, and diagnosis.
An introduction to nanobots
By definition, a nanobot is a miniature robot that is anywhere between a millimeter to a few micrometers in size, which interacts with objects on a nanoscale[3]. Produced by combining inorganic and biological components such as polymers and natural proteins via 3D printing, medical nanobots move through blood vessels and perform specific tasks inside the human body.
Motility is a crucial function performed by nanobots as the purpose of nanobots revolves around the delivery of biological components such as proteins, stem cells[5], and inorganic materials to achieve therapeutic effects. To perform their tasks, nanobots are powered by external sources such as ultrasound, light, or, most commonly, magnetism. Magnetic materials—such as permanent magnets[6]—can be incorporated into nanobots to trigger movement by adjusting the orientation or position of the magnet[4]. Other sources that enable movement include microorganisms like bacteria—which have organelles called flagella that enable physical, motor-like movement—or sperm cells, which are propelled by their tails[3].
Drug delivery and cancer therapy
Drug delivery is a subject of active discussion in relation to the clinical application of nanobots as this technology can enhance the efficacy of drugs. Traditional pharmaceutical drugs, which contain synthetic chemicals designed to treat symptoms, are limited by numerous factors, including the drugs’ short half-life[7] and their rapid clearance from the body. Therefore, they often rely on large therapeutic dosages to produce the desired effect, leading to patients experiencing various side effects and increased toxicity from consuming higher dosages of the drugs[8]. Nanobots present a solution to this problem by providing a motile platform that delivers the exact desired dose of a pharmaceutical component. The aforementioned power sources such as magnetism can be controlled externally to facilitate drug release at the desired location, which is not possible for synthetic drugs that are uncontrollable once administered to the body. Additionally, nanobots, which move autonomously and are small in size, can specifically target areas that are difficult to reach with traditional drug delivery methods—methods that rely on blood circulation alone to reach the desired cell[9].
Targeted delivery of therapeutics is especially well-suited for cancer therapy. Recently, researchers at the Max Planck Institute for Intelligent Systems invented a nanobot with the size, shape, and mobility of a white blood cell, capable of delivering anti-cancer drugs to target cells[10]. Half of the nanobot is covered in a layer of nickel and gold for magnetic properties responsible for propelling the device, and the other half is coated with the cancer drug molecule Doxorubicin. Antibodies for tumor cells are also loaded onto the nanobot, which recognize the target cell by binding to the antigens of the tumor cell. When this technology was tested in an artificial blood vessel using a small magnetic coil, the device demonstrated adequate propulsion to withstand the blood flow and reach the desired cell[10]. Although several limitations to current imaging techniques hinder complete clinical testing, this research marked significant progress in navigating nanobots toward diseased tissues through non-invasive means.
Future research on medical nanobots
Further advancements in the field are essential for the robust development of “embeddable technology.” For instance, the precise mechanisms of nanobot action in drug delivery remain largely unknown, opening opportunities for new studies. Other than the mechanisms, it would be important to acquire the technique for controlling a large number of nanobots for mass drug delivery as the drug “cargo” carried by one nanobot is inadequate for a target tissue much bigger in size. Assessment of the safety of this medical device is another crucial step to facilitate the bench-to-bedside[11] transition. Foreign inorganic materials that compose nanobots should be biodegradable, allowing them to be removed from the body system through a medical device or excretion[8]. They should also be biocompatible, meaning that the nanobots should not cause any immunogenic reactions such as inflammatory responses[8]. Currently, the tests used to ensure short-term and long-term safety are costly and time-consuming, suggesting the need to develop more convenient and straightforward testing procedures.
Artificial intelligence (AI) is expected to play an important role in accelerating research on overcoming the current limitations of medical nanobots. Research has already tested deep learning[12] to successfully estimate the location and orientation of optical nanobots—nanobots controlled by light—but such use is expected to expand. For example, AI is expected to optimize printing time and the needed material for 3D printing of nanobots[3].
* * *
Ray Kurzweil, an American computer scientist and futurist, has predicted that nanobot technology will be applied to human bodies by 2030. Although there are numerous hurdles to overcome, rigorous research being conducted on the subject raises anticipation for the clinical application of nanobots in due time.
[1] Futurism
[2] Artificial cardiac pacemaker: An implantable device used to control the heartbeat of patients experiencing heart failure
[3] Nature
[4] Chemical Reviews
[5] Stem cells: A special group of cells with the capability to divide indefinitely which are used to treat degenerative diseases
[6] Permanent magnet: A magnet that produces its own magnetic field
[7] Half-life: The time taken for a drug’s active substance to reduce to half of the original value
[8] Advanced Science
[9] Pharmaceutics
[10] Science Robotics
[11] Bench-to-bedside: Refers to the process by which research conducted in the laboratory is directly applied for clinical uses
[12] Deep learning: A subset of machine learning based on artificial neural networks